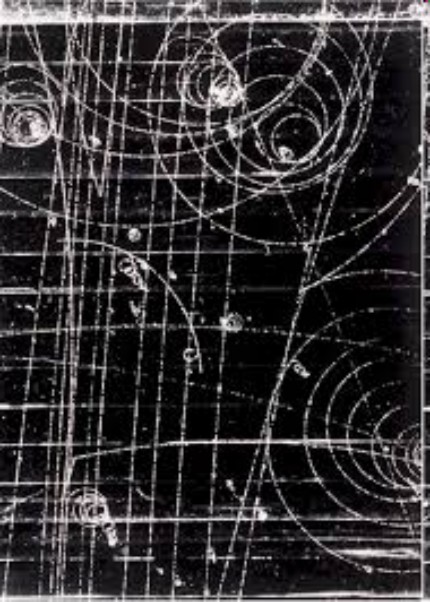
The image at left was taken in from the Brookhaven National Laboratory Bubble Chamber and is perhaps the most famous image of a high-energy particle. Brookhaven’s 33 beV (Billion Electron-Volts) synchrotron particle accelerator sent bursts of 20 -30 K- Mesons into the bubble chamber. In this image, a Kaon (specifically, a K- Meson) entering at the bottom left of the photo is seen colliding with a proton just above the spiraling track. A new visible track is created that slightly veers to the right which is the track created by the Omega-Minus particle. At the point a new track suddenly turns to the right, travelling nearly horizontally across the full image, the Omega-Minus has decayed into a pion and a xi particle.
By 1964, due to a new understanding of sub-atomic particles and advances in particle accelerators, a plethora of new, massive particles had been discovered. The sheer number of particles was troublesome to physicists who looked to discover a structure that contained less "Elementary Particles". Key to this endeavor was grouping particles by their intrinsic properties. Physicists turned to Lie Groups; an extension of Group Theory which had its foundations in the 18th Century as a means to solving polynomial equations with degree higher than four. Group Theory, which modeled the discrete symmetries of algebraic equations led to Lie Groups, which modeled the continuous symmetries of differential equations.
Strong interacting particles - those that interact through the Strong Nuclear Force, the force which keeps atomic nuclei together and the strongest of the Fundamental Forces, all presented similar symmetries. Symmetry implies Conservation and a tenant of Physics is that quantities are conserved. Further, it is believed symmetry is preserved under rotation. Lie Groups provide a mathematical way of connecting similar properties under rotation (three-dimensial space). It also provided a graphical, intuitive means of looking at groups.
The Lie Group SU(2) - Special Unimodular Group of order 2; a 2 x 2 matrix of determinant 1 – provided an invaluable means to making sense of the myriad of particles, but fell short for it allowed too many multiplets (a Proton and a Neutron are so closely related and are considered a multiplet) and failed to accommodate some significant aspects of particle properties.
One of these particle properties was proposed by Murray Gell-Mann to explain a previously discovered mystery of the decay of the K+ Meson. Being a Hadron, 4 times heavier than the Proton, it was expected to decay through the Strong Force with an expected lifetime of 10-23 rather than the observed 10-8 seconds. This short lifetime implied the Weak Force, another Fundamental Force was at work here. But that meant something was inhibiting the Strong Nuclear Force (remember, it is the strongest of all forces). Gell-Mann’s solution was to theorize that there was a Conservation Law that was respected by the Strong Nuclear Force, but not by the decay process. He called this property Strangeness (S), a new Quantum Number; an intrinsic property that takes on the value of +1 or -1, is conserved by the Strong Force but not the Weak, and is reckoned through multiplication. This new property is included in a new quantity called Hypercharge (Y). Y=B+S, where B = the Baryon Number and S = Strangeness, both intrinsic properties.
As SU(2) was insufficient, Physicists turned to SU(3), a 3 x 3 matrix. When the SU(3) group was populated it was apparent something was missing. Gell Mann then proposed a new particle – the Omega-Minus. Through SU(3), Gell-Mann not only predicted the existence of a new particle, but that it was triply-Strange (S = -3), had a Hypercharge of Y = -2, and a predicted mass of 1680 meV.
When the Omega-Minus was discovered it turned out to have a mass of 1672 meV. What a beautiful photo and a beautiful theory…